Introduction
The universe,
our galaxy, our Solar System and the Earth-Moon double planet
system demonstrate some remarkable evidence of intelligent
design. Taken separately, each characteristic is highly
improbable by random chance. When taken together, the
probability is so small as to be impossible - by random chance.
The alternative explanation, design by an intelligent Creator is
a more realistic explanation. Either way, one must admit that we
are a product of a miracle - either a miracle of chance or a
miracle of design. Let's look at a few of the improbable
highlights for the design of the earth and our Solar System.
Unique location
in our galaxy - co-rotation radius
The Sun and
our Solar System have been located in a stable orbit within our
galaxy for the last 4.5 billion years. This orbit lies far from
the center of our galaxy and between the spiral arms. The
stability of our position is possible because the sun is one of
the rare stars that lies within the “galactic co-rotation
radius.” Typically, the stars in our galaxy orbit the center of
the galaxy at a rate that differs from the rate of the trailing
spiral arms. Thus, most stars located between spiral arms do not
remain there for long, but would eventually be swept inside a
spiral arm. Only at a certain precise distance from the galaxy’s
center, the "co-rotation radius," can a star remain in its place
between two spiral arms, orbiting at precisely the same rate as
the galaxy arms rotate around the core ( Mishurov, Y.N. and L.
A. Zenina. 1999. Yes, the Sun is Located Near the Corotation
Circle. Astronomy & Astrophysics 341: 81-85.). Why is it
important that we are not in one of the spiral arms? First, our
location gives us a view of the universe that is unobstructed by
the debris and gases found in the spiral arms. This fact allows
us to visualize what the Bible says, "The heavens declare the
glory of God." If we were within the spiral arms, our view would
be significantly impaired. Second, being outside the spiral arms
puts us in a location that is safer than anywhere else in the
universe. We are removed from the more densely occupied areas,
where stellar interactions can lead to disruption of planetary
orbits. In addition, we are farther from the deadly affects of
supernovae explosions. The 4+ billion year longevity of life on
earth (the time needed to prepare the planet for human
occupation) would not have been possible at most other locations
in our galaxy.
Medvedev, M.V. and A. L.
Melott. 2007. Do extragalactic cosmic rays induce cycles in
fossil diversity? Astrophys. J. 664: 879-889
(arXiv:astro-ph/0602092v3).
Unique
stabilization of the inner solar system
A recent study
reveals some unusual design in our solar system. With the
continuing growth in the capabilities and sophistication of
computer systems, scientists are gaining the ability to model
the dynamics of the Solar System and ask "what if" questions
regarding the presence and size of planets. The presence of
Jupiter is required to allow advanced life to exist on the Earth
(see
below). However, Jupiter's large mass (along with the other
gas giants) has a profound destabilizing effect upon the inner
planets. In the absence of the Earth-moon system, the orbital
period of Jupiter sets up what is called resonance over the
period of 8 million years. This resonance causes the orbits of
Venus and Mercury to become highly eccentric, so much so, that
eventually the orbits become close enough so that there would be
a "strong Mercury-Venus encounter." Such an encounter would
certainly lead to the ejection of Mercury from the Solar System,
and an alteration of the orbit of Venus. In doing the
simulations, the scientists learned that the stabilizing effect
of the Earth-moon requires a planet with at least the mass of
Mars and within 10% of the distance of the Earth from the Sun.
The authors of the study used the term "design" twice in the
conclusion of their study:
Our basic
finding is nevertheless an indication of the need for some
sort of rudimentary "design" in the solar system to ensure
long-term stability. One possible aspect of such "design" is
that long-term stability may require that terrestrial orbits
require a degree of irregularity to "stir" certain
resonances enough so that such resonances cannot persist. (Innanen,
Kimmo, S. Mikkola, and P. Wiegert. 1998. The earth-moon
system and the dynamical stability of the inner solar
system. The Astronomical Journal 116: 2055-2057.)
Unusually
circular orbit of the earth
The unique
arrangement of large and small planetary bodies in the solar
system may be required to ensure the 4+ billion year stability
of the system. In addition, it is readily apparent from the
cycle of ice ages that the earth is at the edge of the life zone
for our star. Although the earth has one of the most stable
orbits among all the planets discovered to date, its periodic
oscillations, including changes in orbital eccentricity, axial
tilt, and a 100,000-year periodic elongation of Earth's orbit,
results in a near freeze over (Kerr, R. 1999. Why the Ice Ages
Don't Keep Time. Science 285: 503-505, and Rial, J.A.
1999. Pacemaking the Ice Ages by Frequency Modulation of Earth's
Orbital Eccentricity. Science 285: 564-568.). According
to Dr. J. E. Chambers, simulations of planetary formation "yield
Earth-like planets with large eccentricities (e ~ 0.15),"
whereas the Earth has an e value of 0.03. He goes on to say,
"Given that climate stability may depend appreciably on e, it
could be no coincidence that we inhabit a planet with an
unusually circular orbit." (Chambers, J. E. 1998. How Special is
Earth's Orbit? American Astronomical Society,
DPS meeting #30, #21.07) With this new information, it seems
very unlikely that stable planetary systems, in which a small
earth-like planet resides in the habitable zone, exist in any
other galaxy in our universe. This does not even consider the
other design parameters that are required for life to exist
anywhere in the universe.
Axial tilt and
eccentricity of orbit
The earth is titled on its
axis at an angle of 23.5°. This is important, because it
accounts for the seasons. Two factors impact the progression of
seasons. The most important is the location of land masses on
the earth. Nearly all of the continental land mass is located in
the Northern Hemisphere. Since land has a higher capacity to
absorb the Sun's energy, the earth is much warmer when the
Northern Hemisphere is pointing towards the Sun. This happens to
be the point at which the earth is farthest from the Sun (the
aphelion of its orbit). If the opposite were true, the seasons
on the earth would be much more severe (hotter summers and
colder winters). For more information, see
Aphelion Away! from the
NASA website.
The presence of
an "impossibly" large moon
The earth has
a huge moon orbiting around it, which scientists now know 1) did
not bulge off due to the earth's high rotational speed and 2)
could not have been captured by the earth's gravity, due to the
moon's large mass. For further explanations, see "The scientific
legacy of Apollo" (2).
The best explanation (other than outright miracle) for the
moon's existence is that a Mars-sized planet crashed into the
earth around 4.25 billion years ago (the age of the Moon). As
you can imagine, the probability of two planets colliding in the
same solar system is extremely remote. Any "normal" collision
would not have resulted in the formation of the moon, since the
ejecta would not have been thrown far enough from the earth to
form the moon. The small planet, before it collided with the
earth, must have had an unusually elliptical orbit (unlike the
orbit of any other planet in the Solar System), which resulted
in a virtual head-on collision. The collision of the small
planet with the earth would have resulted in the ejection of 5
billion cubic miles of the earth's crust and mantle into orbit
around the earth. This ring of material, the theory states,
would have coalesced to form the moon. In addition, the moon is
moving away from the earth (currently at 2 inches per year), as
it has been since its creation. If we calculate backwards we
discover that the moon must have formed just outside the Roche
limit, the point at which an object would be torn apart by the
earth's gravity (7,300 miles above the earth's surface). A
collision which would have ejected material less than the Roche
limit would have formed only rings around the earth. Computer
models show that a collision of a small planet with the earth
must have been very precise in order for any moon to have been
formed at all (coincidence or design?). (see What If the Moon
Didn't Exist?, by Neil F. Comins, professor of Astronomy and
Physics).
Unusually thin
atmosphere
Why is the
moon important to life on earth? The collision of the small
planet with the earth resulted in the ejection of the majority
of the earth's primordial atmosphere. If this collision had not
occurred, we would have had an atmosphere similar to that of
Venus, which is 80 times that of the earth (equivalent to being
one mile beneath the ocean). Such a thick atmosphere on Venus
resulted in a runaway greenhouse affect, leaving a dry planet
with a surface temperature of 800°F. The earth would have
suffered a similar fate if the majority of its primordial
atmosphere had not been ejected into outer space. In fact, the
Earth is 20% more massive than Venus and further away from the
Sun, both factors of which should have lead to a terrestrial
atmosphere much thicker than that of Venus. For some strange
reason, we have a very thin atmosphere - just the right density
to maintain the presence of liquid, solid and gaseous water
necessary to life (coincidence or design?).
Slowing rotation
makes advanced life possible
The moon has
had other beneficial affects on the earth. Scientists now know
that the earth originally had a rotational period of eight
hours. Such a rapid rotational period would have resulted in
surface wind velocities in excess of 500 miles per hour. The
gravitational tug of the moon over the last 4+ billion years has
reduced the rotation period of the earth to 24 hours (likewise,
the gravitational attraction of the earth on the moon has
reduced its rotational period to 29 days). Needless to say,
winds of 500 miles per hour would not be conducive to the
existence of higher life forms (coincidence or design?).
Van-Allen
radiation shield is unique to Earth
Another
fortuitous result of the collision of the Mars-sized planet with
the Earth is the presence of the Earth's large and heavy
metallic core. In fact, the Earth has the highest density of any
of the planets in our Solar System. This large nickel-iron core
is responsible for our large magnetic field. This magnetic field
produces the Van-Allen radiation shield, which protects the
Earth from radiation bombardment. If this shield were not
present, life would not be possible on the Earth. The only other
rocky planet to have any magnetic field is Mercury - but its
field strength is 100 times less than the Earth's. Even Venus,
our sister planet, has no magnetic field. The lack of a magnetic
field on Venus is thought to have resulted in the planet losing
virtually all of its water through stripping by the solar wind
(see
Venus: Express dispatches from Nature). For more
information on the magnetosphere, see NASA's
What is the Magnetosphere? and
Space Weather on Mars. The Van-Allen radiation shield is
a design unique to the Earth (coincidence or design?).
Unique
continental crust and tectonic activity
Recent
evidence tells us that the earth is unique in many ways, even
compared to the other rocky planets in our Solar System. In a
recent study (3),
Dr. Roberta Rudnick says that the earth has a unique continental
crust, which is different from any other planet in our Solar
System (even Venus, our "sister planet"). The mechanisms which
resulted in this unique continental crust is not entirely
certain as she stated, "Perhaps the greatest dilemma facing
those interested in understanding how the continents formed is
their composition." However, the earth's crust is much thinner
(4 km) than that of Venus (30 km). Tectonic processes cannot
happen with such thick plates. If most of the crust of the earth
had not been blown away during the formation of the moon, the
earth would have no continents, but would be completely covered
by water (see
The Moon
And Plate Tectonics: Why We Are Alone from
spacedaily.com). The
tectonic processes which recycle the crust are extremely
important in maintaining life on our planet by recycling
minerals and nutrients (coincidence or design?).
All other
earth-sized planets will be either deserts or waterworlds
Scientists now
know that planets like the earth, with large amounts of both
water and land, are virtually impossible to form. Large planets
do not form continents because the increased gravity prevents
significant mountain and continent formation. Earth-sized
planets completely flood, and any land formed is eroded by the
seas in a short period of time (in the absence of tectonic
activity, which results only from the effects of the formation
of the moon). Smaller planets lack tectonic activity, so would
have no land masses, but would be completely covered with water.
According to Dr. Nick Hoffman of La Trobe University, Melbourne
Australia:
"Around
countless stars in our galaxy, and innumerable galaxies
through space there will surely be Terrestrial planets, yet
they will not be Earth-like. They will not have glistening
Silver Moons orbiting silently through space around them,
but only small dull rocks whizzing in orbit. The worlds will
be, almost without exception, waterworlds." (Venus
- What the Earth would have been like from
spacedaily.com)
Reduction of
greenhouse gases with increasing solar luminosity
Another study
points out the uniqueness of the earth in maintaining
temperatures suitable for life over a period of at least 3.5
billion years. At the formation of the Solar System (about 4.5
billion years ago) the Sun was approximately one third less
luminous than it is now (known from studies of stellar burning
rates). Scientists have postulated that certain greenhouse gases
must have been present at higher concentrations to prevent the
earth from becoming a frozen planet. In a recent study
("Atmospheric carbon dioxide concentrations before 2.2 billion
years ago" published in December, 1995 in Nature) Drs.
Rye, Kuo, and Holland have determined (by sampling ancient
rocks) that carbon dioxide levels could not have been high
enough to compensate for the lower solar luminosity. The
presence of other greenhouse gases, such as ammonia and methane
is also problematical, since it is known that the earth
possessed an oxidative atmosphere even at four billion years ago
(4).
In addition, 1) ammonia is extremely sensitive to solar
ultraviolet radiation and 2) ammonia at levels needed to
influence the earth's temperature would have prevented
photosynthetic organisms from fixing nitrogen (i.e.,
protein,
DNA
and
RNA
synthesis would have been prevented). Fossil evidence indicates
that photosynthetic organisms have been present on the Earth for
at least 3.5 billion years. Methane has similar problems to
ammonia, in that it is sensitive to solar ultraviolet radiation
in an oxidative atmosphere. The problem remains unresolved, but
some unique design must have existed to prevent the Earth from
becoming a planet frozen solid in ice (early on) or a sweltering
inferno (now) (coincidence or design?).
At least part
of the design for the removal of greenhouse gases may have been
answered by a recent study. It seems that life itself (and
rather remarkable life, at that) may have been responsible for
keeping the earth from turning into a scorched planet like
Venus. Scientists have discovered a methane metabolizing Archea
in the extreme pressures of deep sea sediments. It is estimated
that these bacteria-like organisms consume 300 million tons of
methane each year, which prevent the Earth from turning into a
furnace. According to Kai-Uwe Hinrichs, a biogeochemist at the
Woods Hole Oceanographic Institution in Massachusetts and one of
the authors of the study, "If they hadn't been established at
some point in Earth's history, we probably wouldn't be here."
According to an analysis of the study:
"...on
early Earth, these microbes might have been even more
significant. Atmospheric scientists have suggested that
methane levels in the atmosphere may have been 1000 times
higher than they are today, created initially by volcanoes
and later by methane-producing microbes (Science, 25 June
1999, p. 2111). At first, this methane may have been
beneficial, creating a greenhouse effect that kept the
planet from freezing. But if the rise in methane had gone
unchecked, Earth might have become too hot for life, as
Venus is today." (Zimmer, C. 2001. 'Inconceivable' Bugs Eat
Methane on the Ocean Floor.
Science
293: 418-419.)
The need for
Jupiter-sized planets at 5 AU from its star
We have
already discussed the destabilizing effects of large planets in
our Solar System. However, these large bodies are required
for life to exist on the Earth. A recent study implicates
Jupiter as the indirect cause of oceans on the earth. Several
studies have concluded that comets brought water to the earth.
However, there are problems with this theory. The water on the
earth contains 150 ppm deuterium, or heavy hydrogen, which is
five or six times the deuterium-to-hydrogen ratio found in the
sun and in the solar nebula gas. In addition, it's only about a
third of the deuterium-to-hydrogen ratio measured in comets
Halley, Hyakutake, and Hale-Bopp. However, the ratio of
deuterium-to-hydrogen in meteorites is similar to that seen in
the Earth's oceans. Scientists have hypothesized that the
presence of Jupiter sent large amounts of water-containing
meteorites into the inner Solar System soon after it was forming.
It is also possible that Jupiter was also responsible for
sending the Mars-sized planet that formed the moon. What is
unique is that Jupiter-sized planets are not found as far out as
5 AU in other stellar systems. In fact, nearly all large planets
have been found to be closer to their stars than the earth is to
the Sun (which would remove all rocky planets in the habitable
zone from those systems). For more information, see
Only
Solar Systems With Jupiters May Harbor Life (from
spacedaily.com)
"We now
know that these other planetary systems
don't look like the solar system at
all... We also know that the solar
system is special and understand at some
level what makes it special."
(Frederic
Rasio, Professor of Physics and
Astronomy, Northwestern University)
Despite having
been responsible for the shower of meteors that pelted the early
earth, Jupiter is now our
great protector and is responsible for collecting and
ejecting a large proportion of the comets that enter into orbit
around the Sun (e.g., comet
Shoemaker-Levy). Without Jupiter life on Earth at this time
would be difficult or impossible due to the large number of
cometary collisions (approximately 1,000-10,000 times more
collisions) with the Earth (5).
There have been many large planets found around other stars
recently, but none of these planets are far enough away from
their star (most orbit at a position comparable to Mercury) to
stabilize the orbits of planets in the zone that can support
life or protect these inner planets from cometary bombardment
(see
Universally Speaking, Earthlings Share a Nice Neighborhood,
from NSF). The presence of Jupiter-like planets in the universe
is a rare event. According to Dr. Peter D. Ward of the
University of Washington, "All the Jupiters seen today [31 to
date] are bad Jupiters. Ours is the only good one we know of.
And it's got to be good, or you're thrown out into dark space or
into your sun." (See
Rare Earth: Why Complex Life is Uncommon in the Universe,
click for review). Is this coincidence or design?
Conclusion
The following
table ("Uniqueness of the galaxy-sun-earth-moon system for life
support") is based upon the assumption that life is based upon
carbon. As you are probably aware, there has been speculation
that life might be based upon boron or silicon (mainly in
Hollywood productions, such as Star Trek). However, these
elements do not form very long-chained compounds, which would
make any form of life based upon these elements virtually
impossible (6).
Life based
upon carbon requires that water exist in the liquid state (a
very narrow range of 100°C). For practicality, this range
is even more narrow. There are a few bacteria which can exist
near the boiling point, but they are very specialized. Nearly
all other life forms must exist below a temperature of 50°C.
This is the major constraint on the system, which requires
stabile galaxies (spirals only) stabile stars (eliminating all
large or small stars and all binary systems, which most stars
are part of), stabile planetary orbits (orbital eccentricity
must be small), exact rotational characteristics (long
rotational periods will lead to too widely varying temperatures,
short ones to high winds).
The table
below lists the parameters required for a planet to be able to
sustain life. Individually, the probabilities of occurrence of
each parameter are not particularly impressive. The fact that
all of these parameters are found on the Earth is extremely
impressive, indicating an extreme deviation from random chance.
The probability values below are ones obtained from that
observed in the universe as a whole.
Uniqueness of the
Galaxy-Sun-Earth-Moon System for Life Support
- galaxy size
(9)
(p = 0.1)
if too large: infusion of gas and stars would disturb
sun's orbit and ignite deadly galactic eruptions
if too small: infusion of gas would be insufficient
to sustain star formation long enough for life to form
- galaxy type
(7)
(p = 0.1)
if too elliptical: star formation would cease before
sufficient heavy elements formed for life chemistry
if too irregular: radiation exposure would be too
severe (at times) and life-essential heavy elements would
not form
- galaxy location
(9)
(p = 0.1)
if too close to dense galaxy cluster: galaxy would be
gravitationally unstable, hence unsuitable for life
if too close to large galaxy(ies): same result
- supernovae eruptions
(8)
(p = 0.01)
if too close: radiation would exterminate life
if too far: too little "ash" would be available for
rocky planets to form
if too infrequent: same result
if too frequent: radiation would exterminate life
if too soon: too little "ash" would be available for
rocky planets to form
if too late: radiation would exterminate life
- white dwarf binaries
(8)
(p = 0.01)
if too few: insufficient fluorine would exist for
life chemistry
if too many: orbits of life-supportable planets would
be disrupted; life would be exterminated
if too soon: insufficient fluorine would exist for
life chemistry
if too late: fluorine would arrive too late for life
chemistry
- proximity of solar nebula
to a supernova eruption (9)
if farther: insufficient heavy elements would be
attracted for life chemistry
if closer: nebula would be blown apart
- timing of solar nebula
formation relative to supernova eruption
(9)
if earlier: nebula would be blown apart
if later: nebula would not attract enough heavy
elements for life chemistry
- parent star distance from
center of galaxy (9)
(p = 0.2)
if greater: insufficient heavy elements would be
available for rocky planet formation
if lesser: radiation would be too intense for life;
stellar density would disturb planetary orbits, making life
impossible
- parent star distance from
closest spiral arm (9)
(p = 0.1)
if too small: radiation from other stars would be too
intense and the stellar density would disturb orbits of
life-supportable planets
if too great: quantity of heavy elements would be
insufficient for formation of life-supportable planets
- z-axis range of star's
orbit (9)
(p = 0.1)
if too wide: exposure to harmful radiation from
galactic core would be too great
- number of stars in the
planetary system (10)
(p = 0.2)
if more than one: tidal interactions would make the
orbits of life-supportable planets too unstable for life
if fewer than one: no heat source would be available
for life chemistry
- parent star birth date
(9)
(p = 0.2)
if more recent: star burning would still be unstable;
stellar system would contain too many heavy elements for
life chemistry
if less recent: stellar system would contain
insufficient heavy elements for life chemistry
- parent star age
(9)
(p = 0.4)
if older: star's luminosity would be too erratic for
life support
if younger: same result
- parent star mass
(10)
(p = 0.001)
if greater: star's luminosity would be too erratic
and star would burn up too quickly to support life
if lesser: life support zone would be too narrow;
rotation period of life-supportable planet would be too
long; UV radiation would be insufficient for photosynthesis
- parent star metallicity
(9)
(p = 0.05)
if too little: insufficient heavy elements for life
chemistry would exist
if too great: radioactivity would be too intense for
life; heavy element concentrations would be poisonous to
life
- parent star color
(9)
(p = 0.4)
if redder: photosynthetic response would be
insufficient to sustain life
if bluer: same result
- H3+ production
(23)
(p = 0.1)
if too little: simple molecules essential to planet
formation and life chemistry would never form
if too great: planets would form at the wrong time
and place for life
- parent star luminosity
(11)
(p = 0.0001)
if increases too soon: runaway green house effect
would develop
if increases too late: runaway glaciation would
develop
- surface gravity (governs
escape velocity) (12)
(p = 0.001)
if stronger: planet's atmosphere would retain too
much ammonia and methane for life
if weaker: planet's atmosphere would lose too much
water for life
- distance from parent star
(13)
(p = 0.001)
if greater: planet would be too cool for a stable
water cycle
if lesser: planet would be too warm for a stable
water cycle
- inclination of orbit
(22)
(p = 0.5)
if too great: temperature range on the planet's
surface would be too extreme for life
- orbital eccentricity
(9)
(p = 0.3)
if too great: seasonal temperature range would be too
extreme for life
- axial tilt
(9)
(p = 0.3)
if greater: surface temperature differences would be
too great to sustain diverse life-forms
if lesser: same result
- rate of change of axial
tilt (9)
(p = 0.01)
if greater: climatic and temperature changes would be
too extreme for life
- rotation period
(11)
(p = 0.1)
if longer: diurnal temperature differences would be
too great for life
if shorter: atmospheric wind velocities would be too
great for life
- rate of change in
rotation period (14)
(p = 0.05)
if more rapid: change in day-to-night temperature
variation would be too extreme for sustained life
if less rapid: change in day-to-night temperature
variation would be too slow for the development of advanced
life
- planet's age
(9)
(p = 0.1)
if too young: planet would rotate too rapidly for
life
if too old: planet would rotate too slowly for life
- magnetic field
(20)
(p = 0.01)
if stronger: electromagnetic storms would be too
severe
if weaker: planetary surface and ozone layer would be
inadequately protected from hard solar and stellar radiation
- thickness of crust
(15)
(p = 0.01)
if greater: crust would rob atmosphere of oxygen
needed for life
if lesser: volcanic and tectonic activity would be
destructive to life
- albedo (ratio of
reflected light to total amount falling on surface)
(9)
(p = 0.1)
if greater: runaway glaciation would develop
if less: runaway greenhouse effect would develop
- asteroid and comet
collision rates (9)
(p = 0.1)
if greater: ecosystem balances would be destroyed
if less: crust would contain too little of certain
life-essential elements
- mass of body colliding
with primordial earth (9)
(0 = 0.002)
if greater: Earth's orbit and form would be too
greatly disturbed for life
if lesser: Earth's atmosphere would be too thick for
life; moon would be too small to fulfill its life-sustaining
role
- timing of above collision
(9)
(p = 0.05)
if earlier: Earth's atmosphere would be too thick for
life; moon would be too small to fulfill its life-sustaining
role
if later: Earth's atmosphere would be too thin for
life; sun would be too luminous for subsequent life
- oxygen to nitrogen ratio
in atmosphere (25)
(p = 0.1)
if greater: advanced life functions would proceed too
rapidly
if lesser: advanced life functions would proceed too
slowly
- carbon dioxide level in
atmosphere (21)
(p = 0.01)
if greater: runaway greenhouse effect would develop
if less: plants would be unable to maintain efficient
photosynthesis
- water vapor quantity in
atmosphere (9)
(p = 0.01)
if greater: runaway greenhouse effect would develop
if less: rainfall would be too meager for advanced
land life
- atmospheric electric
discharge rate (9)
(p = 0.1)
if greater: fires would be too frequent and
widespread for life
if less: too little nitrogen would be fixed in the
atmosphere
- ozone quantity in
atmosphere (9)
(p = 0.01)
if greater: surface temperatures would be too low for
life; insufficient UV radiation for life
if less: surface temperatures would be too high for
life; UV radiation would be too intense for life
- oxygen quantity in
atmosphere (9)
(p = 0.01)
if greater: plants and hydrocarbons would burn up too
easily, destabilizing Earth's ecosystem
if less: advanced animals would have too little to
breathe
- seismic activity
(16)
(p = 0.1)
if greater: life would be destroyed; ecosystem would
be damaged
if less: nutrients on ocean floors from river runoff
would not be recycled to continents through tectonics; not
enough carbon dioxide would be released from carbonate
buildup
- volcanic activity
(26)
if lower: insufficient amounts of carbon dioxide and
water vapor would be returned to the atmosphere; soil
mineralization would be insufficient for life advanced life
support
if higher: advanced life would be destroyed;
ecosystem would be damaged
- rate of decline in
tectonic activity (26)
(p = 0.1)
if slower: crust conditions would be too unstable for
advanced life
if faster: crust nutrients would be inadequate for
sustained land life
- rate of decline in
volcanic activity (9)
(p = 0.1)
if slower: crust and surface conditions would be
unsuitable for sustained land life
if faster: crust and surface nutrients would be
inadequate for sustained land life
- oceans-to-continents
ratio (11)
(p = 0.2)
if greater: diversity and complexity of life-forms
would be limited
if smaller: same result
- rate of change in
oceans-to-continents ratio (9)
(p = 0.1)
if smaller: land area would be insufficient for
advanced life
if greater: change would be too radical for advanced
life to survive
- distribution of
continents (10)
(p = 0.3)
if too much in the Southern Hemisphere: sea-salt
aerosols would be insufficient to stabilize surface
temperature and water cycle; increased seasonal differences
would limit the available habitats for advanced land life
- frequency and extent of
ice ages (9)
(p = 0.1)
if lesser: Earth's surface would lack fertile valleys
essential for advanced life; mineral concentrations would be
insufficient for advanced life.
if greater: Earth would experience runaway freezing
- soil mineralization
(9)
(p = 0.1)
if nutrient poorer: diversity and complexity of
lifeforms would be limited
if nutrient richer: same result
- gravitational interaction
with a moon (17)
(p = 0.1)
if greater: tidal effects on the oceans, atmosphere,
and rotational period would be too severe for life
if lesser: orbital obliquity changes would cause
climatic instabilities; movement of nutrients and life from
the oceans to the continents and vice versa would be
insufficient for life; magnetic field would be too weak to
protect life from dangerous radiation
- Jupiter distance
(18)
(p = 0.1)
if greater: Jupiter would be unable to protect Earth
from frequent asteroid and comet collisions
if lesser: Jupiter’s gravity would destabilize
Earth's orbit
- Jupiter mass
(19)
(p = 0.1)
if greater: Jupiter’s gravity would destabilize
Earth's orbit 9
if lesser: Jupiter would be unable to protect Earth
from asteroid and comet collisions
- drift in (major) planet
distances (9)
(p = 0.1)
if greater: Earth's orbit would be destabilized
if less: asteroid and comet collisions would be too
frequent for life
- major planet orbital
eccentricities (18)
(p = 0.05)
if greater: Earth's orbit would be pulled out of life
support zone
- major planet orbital
instabilities (9)
(p = 0.1)
if greater: Earth's orbit would be pulled out of life
support zone
- atmospheric pressure
(9)
(p = 0.1)
if smaller: liquid water would evaporate too easily
and condense too infrequently to support life
if greater: inadequate liquid water evaporation to
support life; insufficient sunlight would reach Earth's
surface; insufficient UV radiation would reach Earth's
surface
- atmospheric transparency
(9)
(p = 0.01)
if greater: too broad a range of solar radiation
wavelengths would reach Earth's surface for life support
if lesser: too narrow a range of solar radiation
wavelengths would reach Earth's surface for life support
- chlorine quantity in
atmosphere (9)
(p = 0.1)
if greater: erosion rate and river, lake, and soil
acidity would be too high for most life forms; metabolic
rates would be too high for most life forms
if lesser: erosion rate and river, lake, and soil
acidity would be too low for most life forms; metabolic
rates would be too low for most life forms
- iron quantity in oceans
and soils (9)
(p = 0.1)
if greater: iron poisoning would destroy advanced
life
if lesser: food to support advanced life would be
insufficient
if very small: no life would be possible
- tropospheric ozone
quantity (9)
(p = 0.01)
if greater: advanced animals would experience
respiratory failure; crop yields would be inadequate for
advanced life; ozone-sensitive species would be unable to
survive
if smaller: biochemical smog would hinder or destroy
most life
- stratospheric ozone
quantity (9)
(p = 0.01)
if greater: not enough LTV radiation would reach
Earth's surface to produce food and life-essential vitamins
if lesser: too much LTV radiation would reach Earth's
surface, causing skin cancers and reducing plant growth
- mesospheric ozone
quantity (9)
(p = 0.01)
if greater: circulation and chemistry of mesospheric
gases would disturb relative abundance of life-essential
gases in lower atmosphere
if lesser: same result
- frequency and extent of
forest and grass fires (24)
(p = 0.01)
if greater: advanced life would be impossible
if lesser: accumulation of growth inhibitors,
combined with insufficient nitrification, would make soil
unsuitable for food production
- quantity of soil sulfur
(9)
(p = 0.1)
if greater: plants would be destroyed by sulfur
toxins, soil acidity, and disturbance of the nitrogen cycle
if lesser: plants would die from
protein
deficiency
- biomass to comet-infall
ratio (9)
(p = 0.01)
if greater: greenhouse gases would decline,
triggering runaway freezing
if lesser: greenhouse gases would accumulate,
triggering runaway greenhouse effect
- quantity of sulfur in
planet's core (9)
(p = 0.1)
if greater: solid inner core would never form,
disrupting magnetic field
if smaller: solid inner core formation would begin
too soon, causing it to grow too rapidly and extensively,
disrupting magnetic field
- quantity of sea-salt
aerosols (9)
(p = 0.1)
if greater: too much and too rapid cloud formation
over the oceans would disrupt the climate and atmospheric
temperature balances
if smaller: insufficient cloud formation; hence,
inadequate water cycle; disrupts atmospheric temperature
balances and hence the climate
- dependency factors
(estimate 100,000,000,000)
- longevity requirements
(estimate .00001)
Total Probability = 1:1099
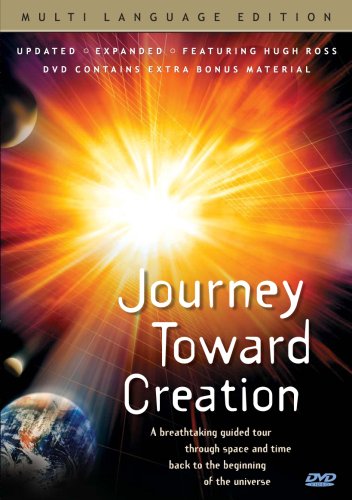